Research
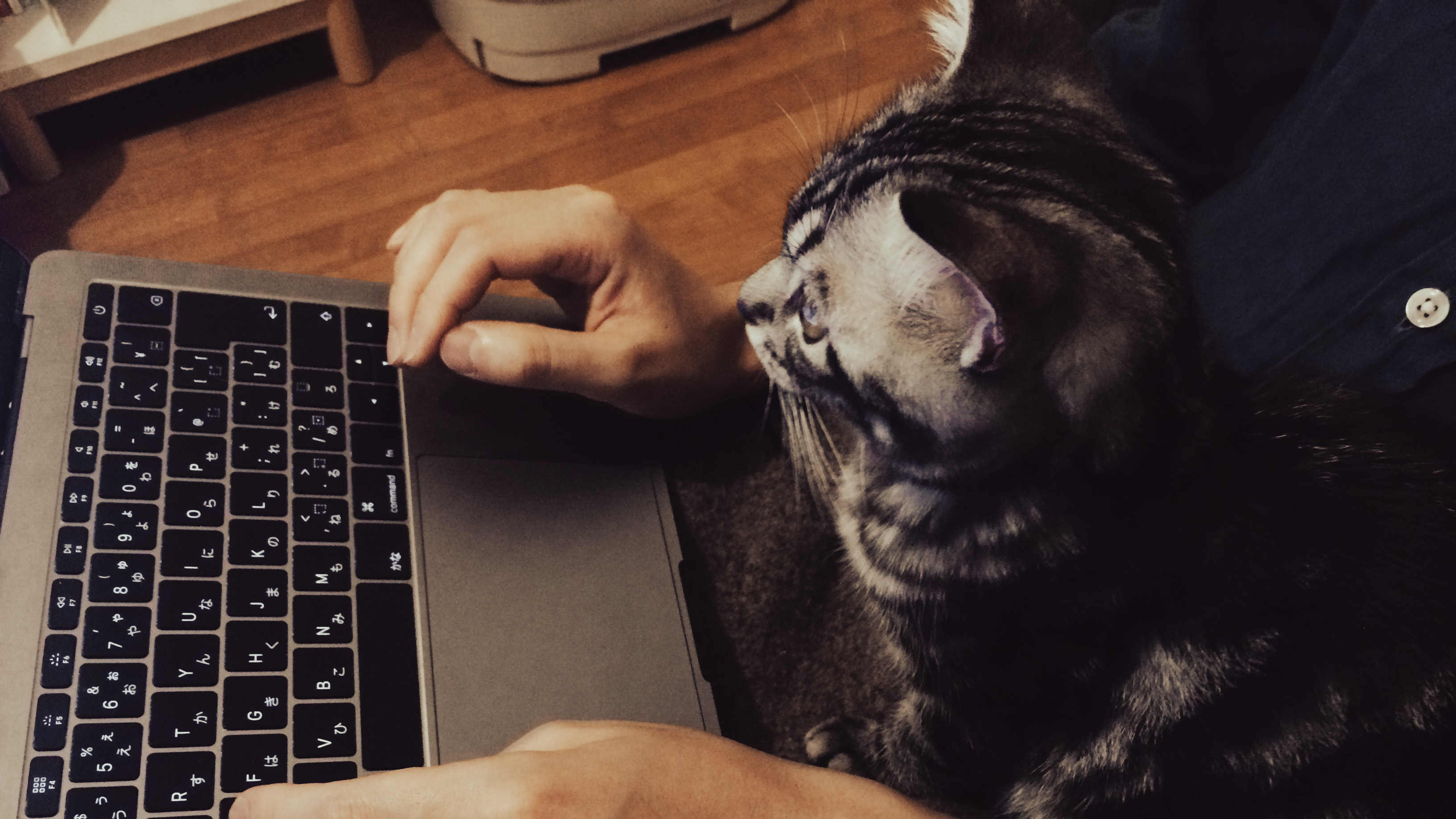
I: Introduction
II: Muon Science
III: Precision Physics Involving Muons
Introduction
The Standard Model of particle physics
Matter is divided into atoms, atoms into nuclei and electrons, nuclei into protons and neutrons, and nucleons into quarks. Particles that cannot be further divided are called elementary particles. The Standard Model is an effective theory of elementary particles and the interactions between them. It describes a framework in which Fermi particles, which constitute matter, and Bose particles, which mediate the electromagnetic, strong, and weak forces. Although the validity of the theory has been verified by various experiments, it has unsolved problems and mysteries, suggesting the existence of unknown physics beyond the model.
素粒子物理学の標準模型
物質は原子からつくられ、原子は原子核と電子から構成されています。原子核は陽子と中性子の組み合わせで成り立っていて、それらはさらにクォークが構成しています。電子やクォークは分割できない基本的な粒子という意味で素粒子と呼ばれます。素粒子物理学における標準模型は素粒子とそれらの間に働く力に関する有効理論で、物質を構成するFermi粒子と力を媒介するBose粒子が電磁気力、強い力、弱い力で相互作用する枠組みを記述します。様々な実験によってその正しさが検証されてきた一方、未解決の問題や謎を抱えており、標準模型を超えた未知の物理の存在が示唆されています。
Elementary particles of the Standard Model. Fermions are matter particle, Bosons are force mediator.
標準模型を構成する素粒子の一覧。Fermi粒子は物質を構成し、Bose粒子は相互作用を伝える。
The Standard Model can explain many phenomena by formulating the nature of elementary particles and the interactions between them. The Higgs mechanism by which matter acquires mass and the breaking of CP symmetry by generational mixing of quarks are also part of the Standard Model. The theoretical framework of the Standard Model was established in 1974, but its experimental verification required a high-energy particle accelerator, which was completed in 2012 with the observation of the Higgs boson by the ATLAS and CMS experiments at LHC.
標準模型は素粒子とそれらの間に働く力のあり方を定式化することで多くの現象を説明できます。物質が質量を獲得するHiggs機構も、クォークの世代間混合によるCP対称性の破れも標準模型の一部です。標準模型の理論的な枠組みは1974年に確立していましたが、その実験的検証には高エネルギーの粒子加速器が必要となり、2012年にLHCにおけるATLAS実験とCMS実験がHiggs粒子を観測したことで完成しました。
A history of the Standard Model, summarized in terms of accelerator energy and the discovery of subatomic particles.
加速器のエネルギーと素粒子の発見という観点からまとめた標準模型の歴史。
Unsolved problems in particle physics
The Standard Model has been very successful in various respects, but there are still mysteries to be solved. The existence of dark matter, neutrino masses, and the hierarchy problem are well known. However, there are many other unsolved problems as well. The Standard Model has 17 elementary particles and 19 parameters, but their origins are unknown. Why are there three generations of quarks and leptons? Why do only weak interactions break the CP symmetry?
素粒子物理学における未解決問題
様々な局面で大きな成功を収めてきた標準模型にも多くの謎が残されています。暗黒物質の存在やニュートリノの質量、階層性問題などがよく知られていますが、ほかにも様々な未解決問題があります。標準模型は17の素粒子と19のパラメーターを持ちますが、その起源はわかっていません。なぜクォークやレプトンは三世代なのでしょうか?なぜ弱い相互作用だけがCP対称性を破るのでしょうか?
Examples of unsolved problems: (left) Composition of matter in the universe; (right) Mass spectrum of elemnetary particles.
未解決問題の例:。(左) 宇宙の構成比。既知の物質はわずか5%。(右) 素粒子の質量。世代によって大きく異なる。
The Standard Model can predict observables that can be experimentally verified precisely. In particular, the perturbation theory of quantum electrodynamics can calculate physical quantities with extremely high precision. By comparing theoretical and experimental results, we can investigate how accurately the Standard Model can describe nature. Some of the experimental results deviate from the theoretical predictions by the Standard Model, and are considered to be signs of new physics.
標準模型は実験的検証が可能な観測量を高精度で予言することができ、中でも量子電磁力学の摂動論はきわめて高い精度で物理量を計算可能です。理論値と実験値とを精密に比較することで、標準模型がどこまで正確に自然を記述できるのか調べることができます。いくつかの実験結果は標準模型による計算値と一定以上の水準で乖離しており、新物理の兆候と目されているものもあります。
Examples of anomalies: (left) the anomalous magnetic moment of electron; (right) the anomalous magnetic moment of muon.
実験値と理論値の不一致の例。(左) 電子の異常磁気能率。(右) ミュオンの異常磁気能率。
Searches for physics beyond the Standard Model
Phenomena that cannot be explained within the scope of the Standard Model provide clues to new physics beyond the exisring theory. The most obvious example is the observation of new elementary particles, where new particles of various types are being searched for in high-energy collider experiments and underground experiments.
Alternatively, quantum effects allow us to tackle areas that are beyond the reach of direct searches. We can search for events with extremely small probability using high-intensity quantum beams or high-intensity electromagnetic fields. Furthermore, it is possible to explore the slightest break in the Standard Model by comparing the results of precise measurements and calculations.
標準模型を超えた物理の探索
標準模型の範疇で説明できない事象は、既知の理論を超えた新たな物理の手がかりを与えてくれます。新物理の存在を未知の素粒子の観測で、様々な種類の新粒子が高エネルギーの衝突型加速器実験や地下素粒子実験で探索されています。
あるいは、直接探索では手の届かない領域に量子効果を介して挑むこともできます。大強度の量子ビームや高強度の電磁場を使ってきわめて低い発生確率しか持たない事象を探索する手法や、高精度で理論値が求められている物理量の測定精度をどこまでも高めていく方法で標準模型のわずかな綻びを探求します。
Four approaches to new physics exploration. Direct and indirect searches are complementary.
新物理を探索する四つの手法。直接探索と間接探索は相補的な関係にある。
Muon Science
Muon and its property
Muons are subatomic particles classified as second-generation charged leptons. They are 200 times heavier than the electron and decay with a lifetime of two microseconds due to weak interactions. It was first discovered as a high-energy cosmic ray, and was later artificially produced using particle accelerators. Muons, like electrons and protons, have an internal degree of freedom called spin. Spin is often likened to the particle's rotation. As for muons, their spin can be imagind as a tiny azimuthal magnet.
ミュオンの性質
ミュオンは第二世代の荷電レプトンに分類される素粒子で、電子より200倍重く、弱い相互作用により2マイクロ秒の寿命で崩壊します。宇宙から飛来する高エネルギーの粒子として発見され、のちに粒子加速器を用いて人工的に生成されるようになりました。ミュオンは電子や陽子と同様にスピンと呼ばれる内部自由度を持っています。スピンはしばしば粒子の自転に喩えられますが、ミュオンに関しては極小の方位磁針のようなものと想像することができます。
Production and decay of muons
In experiments using particle accelerators, a proton beam is irradiated on to a target to produce pions, which decay into a muon and a neutrino. The muons emitted in this reaction are spin polarized. Furthermore, when we use the two-body decay of stopped pions, the muon's kinetic energy is monochromatic. These properties of the muon are of great use as particle beams.
ミュオンの生成と崩壊
粒子加速器を用いた実験では陽子ビームからパイ中間子を生成し、それがミュオンとニュートリノに崩壊する反応が利用されます。このとき放出されるミュオンにはスピンが特定の方向に偏っているという特徴があります。さらに、静止したパイ中間子を使えば生じるミュオンのエネルギーも単一の値になります。これらの性質はミュオンを粒子ビームとして取り出して利用するとき大いに役立ちます。
Production and decay of muon.
ミュオンの生成と崩壊。
Applications of muons
Research using muons can be broadly divided into three directions. (1) experiments to search for unknown physical phenomena by precisely studying the properties of muons as elementary particles, (2) experiments to clarify the magnetic properties of matter and the electronic state of atoms by investigating how muon spin behaves in matter, and (3) experiments to analyze the constituent elements and internal structure of samples by using the interaction between muons and matter. Applications of muons range from basic and applied physics, radiochemistry, life sciences, and archaeological research.
ミュオンの利用
ミュオンを用いた研究には、大きく分けて三つの方向性があります。(1) 素粒子としてのミュオンの性質を精密に調べることを通して未知の物理現象を探索する実験、(2) ミュオンのスピンが物質中でどう振る舞うかを調べて物質の磁気的性質や原子の電子状態を明らかにする実験、 (3) ミュオンと物質の相互作用を利用して試料の構成元素や内部構造を分析する実験です。ミュオンの応用範囲は基礎および応用物理学、放射化学、生命科学、考古学研究まで多岐にわたります。
Precision Physics Involving Muons
Muons for fundamental physics
When looking for new physics using elementary particles, a particle with a larger mass tends to be more susceptible to unknown phenomena. On the other hand, heavier particles are more difficult to produce and have shorter lifetimes, limiting the possible experiments. Muon mass, lifetime, spin, production method, and decay mode strike a fine balance between the pursuit of precision and a search for undiscovered phenomena.
ミュオンと基礎科学
素粒子を探針として新物理を探すとき、質量の大きい粒子ほど未知の現象の影響を受けやすい傾向にあります。一方で、重たい粒子ほど生成が難しく、寿命が短くなるため可能な測定が限定されます。ミュオンは電子より重く、タウ粒子より寿命が長く、スピン偏極しており、スピンと相関した方向に電子を放出します。これらの性質は精密性の追求と未発見の現象の探索を絶妙なバランスで両立し、ミュオンならではのさまざまな実験を可能にします。
Muonic atoms
I am particularly interested in investigating the properties of exotic atoms involving muons to search for physics beyond the Standard Model. Atoms containing muons are virtually nonexistent in nature and are obtained in a facility using particle accelerators. Muons are 207 times heavier than electrons, so they exist much closer to the nucleus in an atom than electrons do. This property provides a special and ideal environment to study the interaction between an elementary particle and a nucleus.
ミュオンがつくる原子
私はミュオンがつくる特殊な原子の性質を詳細に調べて標準模型を超えた新たな物理現象を探索することに興味があります。ミュオンを含む原子は自然界にはほぼ存在せず、粒子加速器を用いた実験施設で作られます。ミュオンは電子と比べて207倍重いので、原子の中では電子と比べてはるかに原子核に近いところに存在します。この性質はミュオンを探針として素粒子と原子核との間の相互作用を調べる特殊かつ理想的な環境を提供します。